Introduction
Type 2 diabetes mellitus can be a difficult disease to live with and can severely affect one’s quality of life. Diabetes mellitus is a chronic condition in which your body cannot regulate your blood glucose levels, the two main types being type 1 and type 2. These are due to either an inability to produce insulin (type 1) or when the insulin produced is ineffective (type 2). Type 2 diabetes, or non-insulin dependent diabetes mellitus, can occur as a result of lifestyle factors, such as diet and obesity. These lead to insulin resistance or the inability to produce enough insulin as necessary. Currently, there are 4.1 million people in the UK with diabetes, with 90% of these cases due to type 2 diabetes. It is estimated that 1 in 10 adults will develop type 2 diabetes by 2030 (Lacobucci, 2021)
One treatment for type 2 diabetes is the use of sulfonylureas – a group of oral drugs with hypoglycaemic effects (ability to lower blood glucose levels). Since their discovery in the 1940’s, medicinal chemists have changed the structure of these drugs, to make them more effective for clinical use. These modifications have led to more favourable properties in metabolism, potency, efficacy and safety, which have made the drugs a more effective, safe and convenient treatment for type 2 diabetes mellitus. These will be discussed later on in the article.
This article will explain the chemistry of sulfonylureas, the pharmacology behind them and how they have changed over time to make them more effective in the treatment of type 2 diabetes mellitus.
Type 2 Diabetes Mellitus Cause
Type 2 diabetes occurs when there is a deficiency in insulin secretion by the β-cells in the pancreas, or when cells develop a resistance to insulin action (Galicia-Garcia, et al., 2020). This is usually due to obesity and an unhealthy lifestyle, including lack of exercise, and a high fatty and sugar diet. Insulin is a peptide hormone that is secreted by β-cells in the pancreas. It is responsible for lowering blood glucose levels by stimulating the conversion of glucose in the blood into glycogen to be stored in muscle, fat, and liver cells. When there is a deficiency or resistance of insulin it leads to hyperglycaemia (high blood glucose levels), due to the reduced ability to convert glucose into glycogen. This would lead to symptoms such as vomiting, dehydration, confusion, increased thirst, and blurred vision to name a few.
Physiology Behind Insulin Secretion and Structure
To understand the pharmacology of the sulfonylurea compounds,one must first understand the physiology behind the secretion of insulin.
As stated above, insulin is a peptide hormone, so it is made from a polypeptide chain. Transcription of the insulin gene (found on chromosome 11) occurs and the resulting mRNA strands are translated to produce two peptide chains. These chains are held together in a quaternary structure by two disulfide bonds to form the hormone insulin (Brange & Langkjoer, 1993).
Insulin secretion must be tightly controlled to maintain efficient glucose homeostasis. To do so, the secretion of insulin is regulated precisely to meet its demand. The β-cells of the pancreas contain glucose transporter 2, a carrier protein that allows facilitated diffusion of glucose molecules across a cell membrane. These transporters allow glucose to be detected and enter the β-cells. Upon cytoplasmic glucose levels rising, the pancreatic β-cells respond by increasing oxidative metabolism, leading to increased ATP in the cytoplasm (Fridlyand & Philipson, 2010). The ATP in the cytoplasm of the β-cells, can bind to ATP sensitive K+ channels on the cell membrane, causing them to close. This leads to a build up of K+ ions within the cell as they are unable to leave the cell, leading to the depolarisation of the cell. The increasing positive membrane potential, leads to the opening of voltage gated Ca2+ channels, leading to an influx of Ca2+ ions. This further depolarises the cell, which triggers the release of insulin from the cell, packaged in secretory vesicles, by exocytosis (Fu, et al., 2013).
Pharmacology of Sulfonylureas
Sulfonylurea’s act inside the pancreatic β-cells. On the ATP sensitive K+ channel, there are sulfonylurea receptors to which the drug binds, causing them to close. The cascade of events that follows leads to the release of insulin by the pancreatic β-cell. This mimics the activity that occurs when glucose is taken into the cell, as mentioned earlier. (Panten, et al., 1996). (possibly delete this instead as it is repeated)
This process allows more insulin to be released, to lower blood glucose levels when insufficient insulin is produced naturally. Sulfonylureas are only effective in type 2 diabetes, since insulin production is not impaired (as in type 1 diabetes), rather the release of or resistance to insulin is affected.
Common Chemistry of all Sulfonylureas
All the sulfonylurea drugs are characterised by their common sulfonylurea group. This functional group allows this unique group of compounds to bind to SUR on ATP sensitive K+ channels, giving it its hypoglycaemic properties. The common structure of sulfonylureas is shown in figure 1 (Fvasconcellos, 2011), with the blue R groups indicating replaceable side chains, which fluctuates between each drug development over time giving slightly different properties between the drugs. Over time, scientists have improved the drugs efficacy by changing the side compounds. Additionally, scientific research has led to development of other drugs from the same pharmacological group, but with altered side chains (again, giving them different properties) which have also improved the efficacy of the drug. These changes have altered properties of the drug such as potency, metabolism, half-life, tolerance and safety, to make the drug more effective for clinical use.
History and development of the drugs and their chemical structure
Sulfanilamide and IPTD
In 1935, a French research term discovered the active chemical in the antibiotic prontosil, known as sulfanilamide (Sorkhy & Ghemrawi, 2020). Sulfanilamide was found to be a poor antibiotic and so derivatives of it were synthesised and tested. These compounds, such as such as p-amino-sulfonamide-isopropylthiodiazole (IPTD), which was used as an antibiotic for the treatment of typhoid in 1942, revealed unexpected hypoglycaemic side effects. These were discovered by French physician, Marcel Janbon (Quianzon & Cheikh, 2012). However, scientists could not identify how these side effects were caused.
In 1946, Auguste Loubatières, investigated the effect of IPTD on dogs. He administrated the drug to fully pancreatectomized and partially pancreatectomized dogs and found that the drug was ineffective in the fully pancreatectomized ones but effective in the partially pancreatectomized ones. This later lead to his conclusion that the drugs’ hypoglycaemic property was due to its ability to stimulate insulin secretion directly in the pancreatic β-cells (Loubatières-Mariani, 2007).
Carbutamide
The first sulfonylurea to be marketed as a drug for diabetes was Carbutamide. It was synthesised in East Germany by Ernst Carstens and in the early 1950’s, clinical trials for this sulfanilamide derivative Carbutamide were carried out, by Hellmuth Kleinsorge, for the treatment of urinary tract infections. However, during treatment, side effects of hypoglycaemia were also noted (Kleinsorge, 1998) – similar to those experienced by patients treated with IPTD for typhoid in 1942.
These findings were presented to Erich Haak, of the East German Ministry of Health, in 1952, which ultimately culminated in the ban of the drug. Haak later moved to West Germany where he patented the drug to be tested for antibacterial use, without disclosing the side effects of hypoglycaemia. Karl Joachim Fuchs, a doctor who was part of this drug testing, noticed symptoms of ravenous hunger and euphoria upon taking the drug himself, which were found to be due to hypoglycaemia. Following this, studies were undertaken, and a general conclusion was that Carbutamide was most effective in people over 45 years of age, who had had diabetes for less than 5–10 years and had not used insulin for more than 1–2 years (Tattersall, 2008). The use of Carbutamide was short lived as it was found to have fatal side effects in a small number of people, including toxic effects on bone marrow (National Center for Biotechnology, 2005).
The structure of Carbutamide is shown in figure 2 (Anon., 2021). It can be seen, attached to the benzene ring on the left-hand side of the sulfonylurea functional group, can be seen an amine group. Attached to a second amine group on the right side of the functional group is a four-carbon chain. As mentioned previously, it is the sulfonylurea functional group that gives rise to the drugs hypoglycaemic effects. This is the first drug to contain the sulfonylurea functional group (seen in figure 1) and the beginning of many discoveries into the treatment of non-insulin dependent diabetes mellitus.

Tolbutamide
After the discovery of the fatal side effects of Carbutamide, the next sulfonylurea drug to be synthesised was Tolbutamide; it was one of the first sulfonylureas to be marketed for controlling of type 2 diabetes, in 1956 in Germany (Quianzon & Cheikh, 2012). There were minimal changes to the chemical structure in this next development of the sulfonylureas. The amine group on the left hand side of Carbutamide was swapped for a methyl group to give Tolbutamide, shown in figure 3 (Anon., 2021), which helped reduce the toxicity of the drug. However, as a result tolbutamide was subsequently being metabolised too quickly (Monash University, 2021), which led to low levels of the (active) drug in the blood. The drugs efficacy was therefore lower than expected, resulting in it having to be administered twice a day, which was an inconvenience for patients.
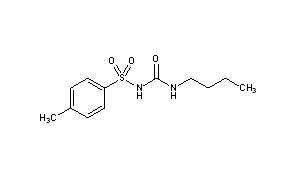
Chlorpropamide
It was soon discovered that the methyl group attached to the benzene ring in Tolbutamide was the site of its metabolism (Monash University, 2021) and so it was replaced by medicinal chemists with a chlorine atom in the next drug, Chlorpropamide (see figure 4 ), (Anon., 2021). This helped reduce metabolism, giving the drug a longer half-life, so it was not cleared as quickly from the body. Indeed, a University of Michigan study found that chlorpropamide serum concentration declined from about 21 mg/100ml at 15 min to about 18 mg/100ml at 6 hours, whereas the tolbutamide serum concentration fell more rapidly from about 20 mg mg/100ml at 15 min to about 8 mg/100ml at 6 hours. Therefore, it could be seen that under experimental conditions, tolbutamide disappeared from the blood approximately 8 times faster than chlorpropamide (Knauff, et al., 1959). This would mean less frequent dosing with chlorpropamide, which would make the drug much more convenient for patients to treat type 2 diabetes. However, further research subsequently revealed that, due to the longer half-life of chlorpropamide, the hypoglycaemic effects were compounded and lasted longer than previously expected (Sola, et al., 2015). This meant that Chlorpropamide could not be administered for the safe treatment of type 2 diabetes.
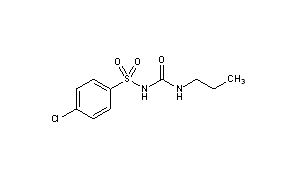
Glibenclamide
Glibenclamide is the first of what is known as the second-generation sulfonylureas. Introduced for use in 1984, these mainly replaced the first-generation drugs (Carbutamide, Tolbutamide, Chlorpropamide etc) in routine use to treat type 2 diabetes. Due to their increased potency and shorter half-lives, lower doses of these drugs could be administered and only had to be taken once a day (Tran, 2020). These second-generation sulfonylureas have a more hydrophobic right-hand side, which results in an increase in their hypoglycaemic potency (Skillman & Feldman, 1981). In Glibenclamide, the left-hand side of the drug changed drastically from chlorpropamide, as seen in figure 5 (Anon., 2021). This suggested to medicinal chemists, an innumerable number of possible changes that could be made to the drug, simply by changing the left and right-hand sides, resulting in better potency, safety, efficacy and convenience (Monash University, 2021). Consequently, the metabolism of the drug varied between patients, and this in addition to increased hypoglycaemia and increased incidence of Cardiovascular events (Scheen, 2021), meant that the drug is not a first choice in recommendation to treat type 2 diabetes.

Glipizide
Glipizide, figure 6 (Anon., 2021), shares the same hydrophobic structure on the right-hand side as Glibenclamide, however a few changes have been made to the left-hand group, resulting in faster metabolism. Although it has similar potency to that of Glibenclamide; however, the duration of its effects was found to be much shorter (Brogden, et al., 1979). Glipizide has the lowest elimination half-life of all the sulfonylureas, reducing the risk of the long-lasting hypoglycaemic side effects found in previous developments (Anon., 2022).

Gliclazide
Gliclazide is the most common sulfonylurea used in current medicine for the treatment of non-insulin dependent diabetes mellitus; it is part of the World Health Organisation’s most recent list of essential medicines (World Health Organisation, 2021). The chemical structure of Gliclazide can be seen in figure 7 (Anon., 2021). Fascinatingly, medicinal chemists returned to the use of a methyl group on the left-hand side of the drug, which was last seen in Tolbutamide. As mentioned before, the left-hand group on the drug, attached to the benzene ring, is responsible for the metabolism of the compound. Returning to the use of a methyl group, allows for a faster metabolism of the drug, which helped to remove the unwanted longer hypoglycaemic side effects, especially for use with elderly patients (Monash University, 2021). The right-hand group of gliclazide is comprised of two hydrophobic rings which, as mentioned previously, are responsible for its increased potency. Gliclazide has also been shown to be one of the most effective sulfonylureas. According to Harrower, three studies carried out concluded that gliclazide is a potent hypoglycaemic agent, which compares favourably with others of its type (Harrower, 1991).
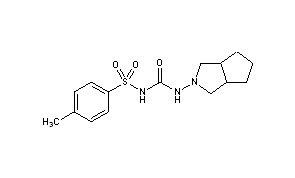
Conclusion
Sulfonylureas are one of several groups of drugs used to treat type 2 diabetes. Through research and trials, they have developed significantly over time, to become one of the most prescribed medications in the effective treatment of type 2 diabetes.
The sulfonylureas discussed above represent significant developments in physiology and pharmacology of the group, since their initial discovery. Other sulfonylurea drugs have been synthesised and tested over the years, such as tolazamide and acetohexamide, however these are less commonly prescribed because of their disadvantages in potency and safety. The discovery of the ability to modify the left and right sides of the drug’s common structure has led to many new forms within this class, with varying properties in potency, metabolism, efficacy, and safety. The experimentation of the chemical structures over time has led to the production of more effective treatments for the disease. Currently, Glipizide and Gliclazide are the two most commonly prescribed sulfonylureas, due to their high potencies and suitable half-lives, while maintaining minimal side effects. These now provide an effective treatment in helping reduce the symptoms of type 2 diabetes and thus improving quality of life for those suffering with the disease.
AliMahdi Meghiji, Youth Medical Journal 2022
References
Anon., 2021. Carbutamide. [Online]
Available at: https://www.drugfuture.com/chemdata/carbutamide.html
[Accessed 27 March 2022].
Anon., 2021. Chlorpropamide. [Online]
Available at: https://www.drugfuture.com/chemdata/chlorpropamide.html
[Accessed 29 March 2022].
Anon., 2021. Gliclazide. [Online]
Available at: https://www.drugfuture.com/chemdata/gliclazide.html
[Accessed 30 March 2022].
Anon., 2021. Glipizide. [Online]
Available at: https://www.drugfuture.com/chemdata/glipizide.html
[Accessed 29 March 2022].
Anon., 2021. Glyburide. [Online]
Available at: https://www.drugfuture.com/chemdata/glyburide.html
[Accessed 29 March 2022].
Anon., 2021. Tolbutamide. [Online]
Available at: https://www.drugfuture.com/chemdata/tolbutamide.html
[Accessed 29 March 2022].
Anon., 2022. Glipizide. [Online]
Available at: https://pharmaoffer.com/api-excipient-supplier/glipizide#:~:text=About%20Glipizide&text=It%20was%20first%20introduced%20in,glucose%2Dlowering%20therapy%20following%20metformin.
[Accessed 29 March 2022].
Brange, J. & Langkjoer, L., 1993. Insulin structure and stability, Bagsvaerd: Novo Research Institute.
Brogden, R. N. et al., 1979. Glipizide: a review of its pharmacological properties and therapeutic use. Drugs , 18(5), pp. 329-353.
Fridlyand, L. E. & Philipson, L. H., 2010. Glucose sensing in the pancreatic beta cell: a computational systems analysis. Theoretical Biology and Medical Modelling, 7(1), p. Article 15.
Fu, Z., Gilbert, E. R. & Liu, D., 2013. Regulation of Insulin Synthesis and Secretion and Pancreatic Beta-Cell Dysfunction in Diabetes. Current Diabetes Reviews, 9(1), pp. 25-53.
Fvasconcellos, 2011. General structural formula of a sulfonylurea, highlighting the functional group that gives the class its name and the side chains that distinguish its various members., s.l.: Wikipedia.
Galicia-Garcia, U. et al., 2020. Pathophysiology of Type 2 Diabetes Mellitus. International Journal of Molecular Sciences, 30 August, 21(17), p. 2.
Harrower, A. D., 1991. Efficacy of gliclazide in comparison with other sulphonylureas in the treatment of NIDDM. Diabetes research and clinical practice , 14(2), pp. 65-67.
Kent, M., . Advanced Biology. ed. (): Oxford University Press.
Kleinsorge, H., 1998. Carbutamide–the first oral antidiabetic. A retrospect. Experimental and clinical endocrinology & diabetes : official journal, German Society of Endocrinology [and] German Diabetes Association, 106(2), pp. 149-151.
Knauff, R. E., Fajans, S. S., Ramirez, E. & Conn, J. W., 1959. Metabolic studies of chlorpropamide in normal men and in diabetic subjects.. Annals of the New York Academy of Sciences , 74(3), pp. 603-617.
Lacobucci, G., 2021. The British Medical Journal. [Online]
Available at: https://www.bmj.com/content/375/bmj.n2453
[Accessed 2 March 2022].
Loubatières-Mariani, M.-M., 2007. The discovery of hypoglycemic sulfonamides. Journal de la Société de Biologie, 201(-), pp. 121-125.
Monash University, 2021. The Science of Medicines MOOC, Melbourne: Future Learn.
National Center for Biotechnology, 2005. PubChem Compound Summary for CID 9564, Carbutamide. [Online]
Available at: https://pubchem.ncbi.nlm.nih.gov/compound/Carbutamide
[Accessed 18 March 2022].
Panten, U., Schwanstecher, M. & Schwanstecher, C., 1996. Sulfonylurea receptors and mechanism of sulfonylurea action.. Experimental and clinical endocrinology & diabetes : official journal, German Society of Endocrinology [and] German Diabetes Association, 104(1), pp. 1-9.
Quianzon, C. C. L. & Cheikh, I. E., 2012. History of current non-insulin medications for diabetes mellitus. Journal of Community Hospital Internal Medicine Perspectives , 2(3), p. 19081.
Scheen, A. J., 2021. Sulphonylureas in the management of type 2 diabetes: To be or not to be?. Diabetes Epidemiology and Management, Volume 1, p. Article 100002.
Skillman, T. G. & Feldman, J. M., 1981. The pharmacology of sulfonylureas. The American journal of medicine, 70(2), pp. 361-372.
Sola, D. et al., 2015. Sulfonylureas and their use in clinical practice. Archives of medical science , 11(4), pp. 840-848.
Sorkhy, M. A. & Ghemrawi, R., 2020. Treatment: Projected Modalities for Antimicrobial Intervention. Microbiomics – Dimensions, Applications, and Translational Implications of Human and Environmental Microbiome Research, -(-), pp. 279-298.
Tattersall, R., 2008. Discovery of the sulphonylureas. TATTERSALL’S TALES, 7(2), p. 74.
Tran, D., 2020. Oral Hypoglycemic Agent Toxicity. [Online]
Available at: https://emedicine.medscape.com/article/1010629-overview#a1
[Accessed 27 March 2022].
World Health Organisation, 2021. WHO model list of essential medicines – 22nd list, 2021. [Online]
Available at: https://www.who.int/publications/i/item/WHO-MHP-HPS-EML-2021.02
[Accessed 30 March 2022].
Leave a comment